Running Head: Response of Pulmonary Vasculature to Endoscopic Lung Volume Reduction
Funding Support: This research has been supported by National Institute of Health Grants 5T32HL007633-29 (F.N.R.), 1R01HL116931-01 (R.S.J.E./G.R.W) and 1K01HL118714 (A.A.D.)
Date of Acceptance: April 2, 2015
Abbreviations: computed tomography, CT; cross sectional area, CSA; volume of blood vessels with cross sectional area of less than or equal to 5mm2, BV5; total blood vessel volume, TBV; pulmonary function tests, PFTs; total lung capacity, TLC; residual volume, RV; diffusion capacity of lung for carbon monoxide, DLCO; forced expiratory volume in 1 second, FEV1; right lower lobe, RLL; right upper lobe, RUL
Citation: Rahaghi FN, Come CE, Ross J, et al. Morphologic response of the pulmonary vasculature to endoscopic lung volume reduction. Chronic Obstr Pulm Dis. 2015; 2(3): 214-222. doi: http://doi.org/10.15326/jcopdf.2.3.2014.0164
Introduction
Emphysema is characterized by destruction of the lung parenchyma with resultant loss of lung elastic recoil, expiratory airflow obstruction, and subsequent hyperinflation. Therapies for this disease include pharmacologic deflation through the administration of inhaled bronchodilators as well as more targeted interventions such as lung volume reduction surgery and analogous endoscopic procedures. These techniques have been shown to have some benefits in patients with advanced emphysema,1-5 leading to improvements in pulmonary function and exercise capacity. The basis for functional improvement following targeted lung deflation is lobar interdependence. Regional deflation of diseased parenchyma either through surgical excision or the induction of atelectasis results in the expansion of lung remote to the treatment site, a finding which has been observed on serial computed tomography (CT) chest imaging.2 Little is known, however, about the relationship between change in lobar volume and change in vascular morphology at these untreated sites.
An initial concern for lung volume reduction was that removal of a part of the vascular bed would result in worsening pulmonary hemodynamics in patients who likely already have some degree of vascular insufficiency. However, lung volume reduction has not been found to lead to increased pulmonary arterial pressures,6,7 possibly due to vascular decompression in the now deflated lung, a process that may be evident on a CT scan.7 Studies have also shown increased heart volume and improved right ventricular function after lung volume reduction.8,9 It has further been demonstrated that oxygen pulse (a surrogate for left ventricular stroke volume) improves after lung volume reduction and is directly related to deflation.10 Thus, improvement in cardiac function may also help preserve pulmonary hemodynamics after lung volume reduction.
Using data obtained from a cohort of patients who underwent sealant-based endoscopic lung volume reduction as part of a clinical trial, we sought to determine if post procedural changes in vascular morphology could be detected on a CT scan. Specifically, metrics of small vascular density were studied, as these metrics have been shown to correlate with perfusion.11 Further, we aimed to determine whether change in lobar volume relates to change in blood vessel volume in non-treated lobes, and whether the change in lobar volume differentially affects the arterial or venous phases of the pulmonary circulation. In studying this question, we also aimed to show the potential utility of three-dimensional vascular reconstruction as a way to study the effect of interventions on the pulmonary vasculature in patients with emphysema.
Materials and Methods
Twenty individuals who underwent endoscopic lung volume reduction with the Emphysematous Sealant System (Aeris Therapeutics, Inc) as part of a prospective study1 were reviewed for availability of sufficient imaging. In that study, 20 patients with severe airflow obstruction, hyperinflation, and either upper lobe predominant or homogeneous emphysema underwent treatment at 2 subsegments in each upper lobe. Treatment involved endoscopic injection of sealant leading to collapse of the targeted lobe. For our investigation, we considered imaging adequate if the pre- and post-treatment volumetric CT scans had similar image resolution (with slice thickness of less than 1 mm and the same for both slices), acquisition, and reconstruction algorithms. Significant parenchymal remodeling was noted in the upper lobes, including scarring and atelectasis, and thus only the vasculature in the lower lobes was examined. The lungs and lobes were segmented,12 and the intraparenchymal vascular reconstruction was performed as described previously.13-15 The right lower lobe was then selected for additional manual labeling of the arteries and veins. This lobe was selected as prior imaging studies have generally focused on the right lung, in part due to presence of cardiac motion artifact on the left.
The blood vessel volume of the intraparenchymal pulmonary vasculature was calculated for each lower lobe. The total blood vessel volume (TBV) was then graphically depicted as a function of vascular cross sectional area (CSA).15 From these data, the blood vessel volume for all vessels of a select range of cross sectional areas was also calculated16-18 by multiplying the length of each detected segment by its cross sectional area. For example, the small blood vessel volume (BV5) was the volume of all blood vessels with a cross sectional area less than 5mm2. Total blood vessel volume was the volume computed from all pulmonary vasculature with cross sectional area of 100mm2. The ratios of BV5 to TBV and lobar volume for the corresponding lobe were calculated. The vascular reconstructions for the right lower lobe underwent additional visual inspection and each vessel segment was then given a label of artery or vein.
Statistical analyses were performed using SAS 9.3 (SAS institute, Cary, North Carolina). Continuous variables are presented as medians and interquartile ranges and dichotomous variables as numbers and proportions. The Wilcoxon Signed Rank test was used to evaluate changes in vasculature and lobar volumes between baseline and week 12. Spearman correlation coefficients were used to evaluate relationships between variables. P values of less than 0.05 were considered significant.
Results
Twelve of the 20 study participants had available volumetric CT scans at study enrollment and 12 weeks post treatment that were of sufficient quality for vascular reconstruction. The imaging methods used for the acquisition of each participant are shown in the online supplement Table 1. The participant’s baseline demographics, functional status, and pulmonary function tests (PFTs) are shown in Table 1. Baseline PFTs were available for all participants and follow-up test results were available for 10 individuals. In the 10 individuals with longitudinal measures of lung function, there was a statistically significant increase in forced expiratory volume in 1 second (FEV1) and statistically significant decreases in total lung capacity (TLC) and residual volume (RV) post treatment. No change in diffusion capacity of the lung for carbon monoxide (DLCO) was noted (Table 1).
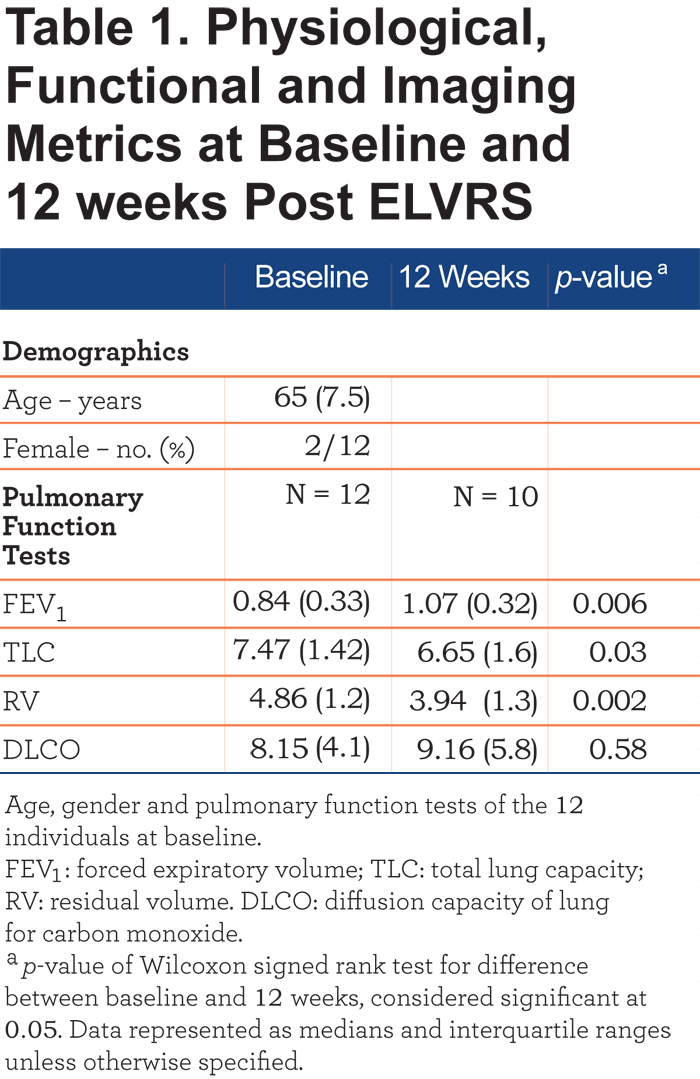
Baseline and 12-week follow-up CT scans were successfully segmented by lobes. There was a significant decrease in the volume of the treated upper lobes (Table 2) and an increase in the volume of the untreated lower lobes. Overall there were decreases in total and right lung volumes with a trend toward decrease in left lung volume (p = 0.09).
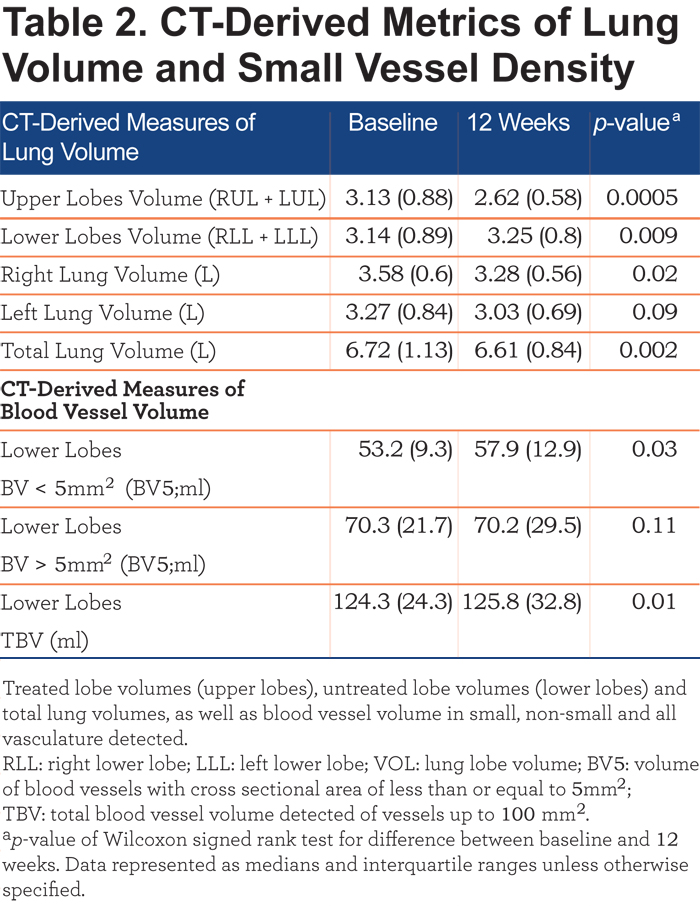
Lower lobe vasculature (right and left) was reconstructed for all 12 individuals. An example of this reconstruction is shown in Figure 1.
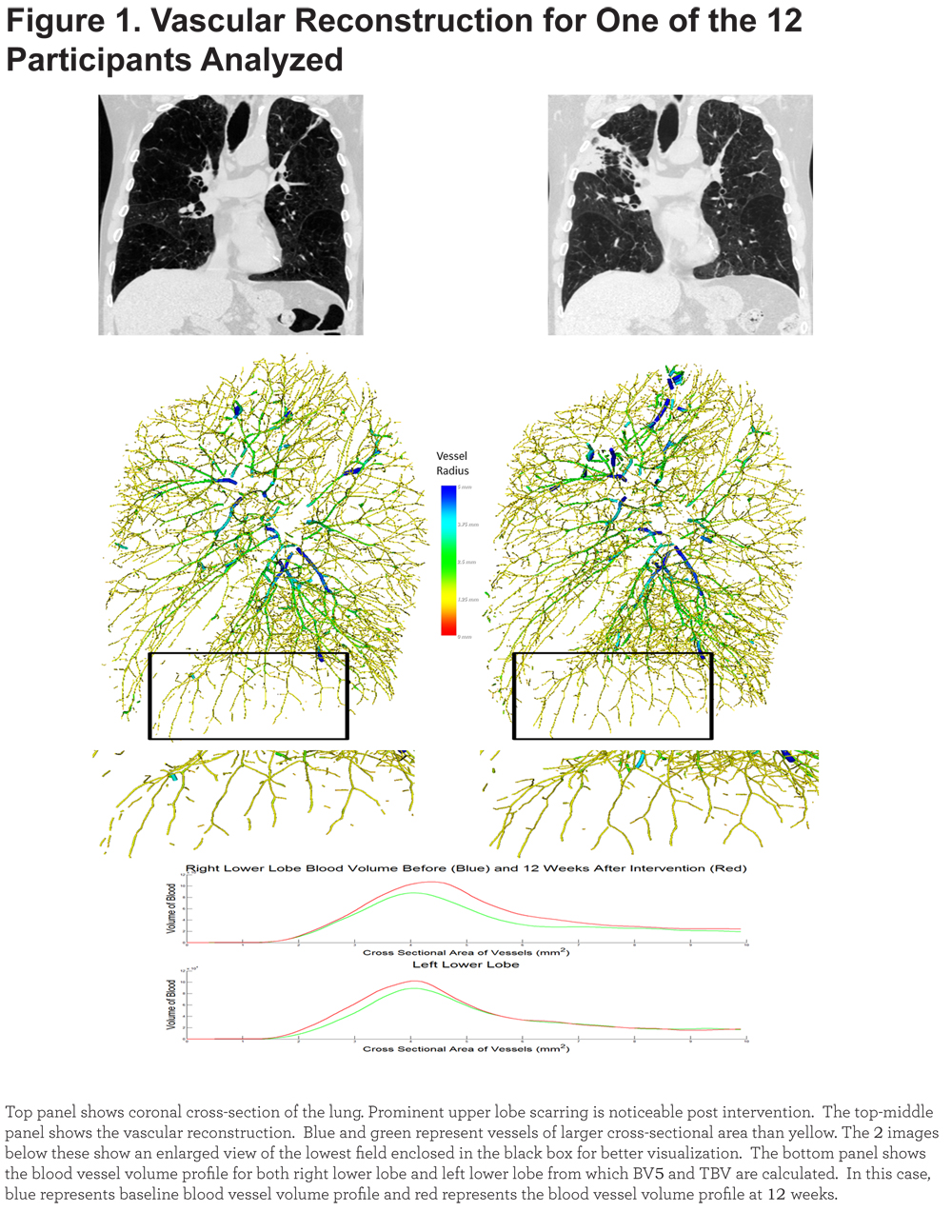
Quantitative assessment of lower lobe vascular morphology revealed an increase in BV5 and TBV post treatment. Treatment had no effect on the volume of blood vessels greater than 5mm2 (Table 2). Lobe and BV5 volume for each of the 12 individuals are shown in Figure 2.
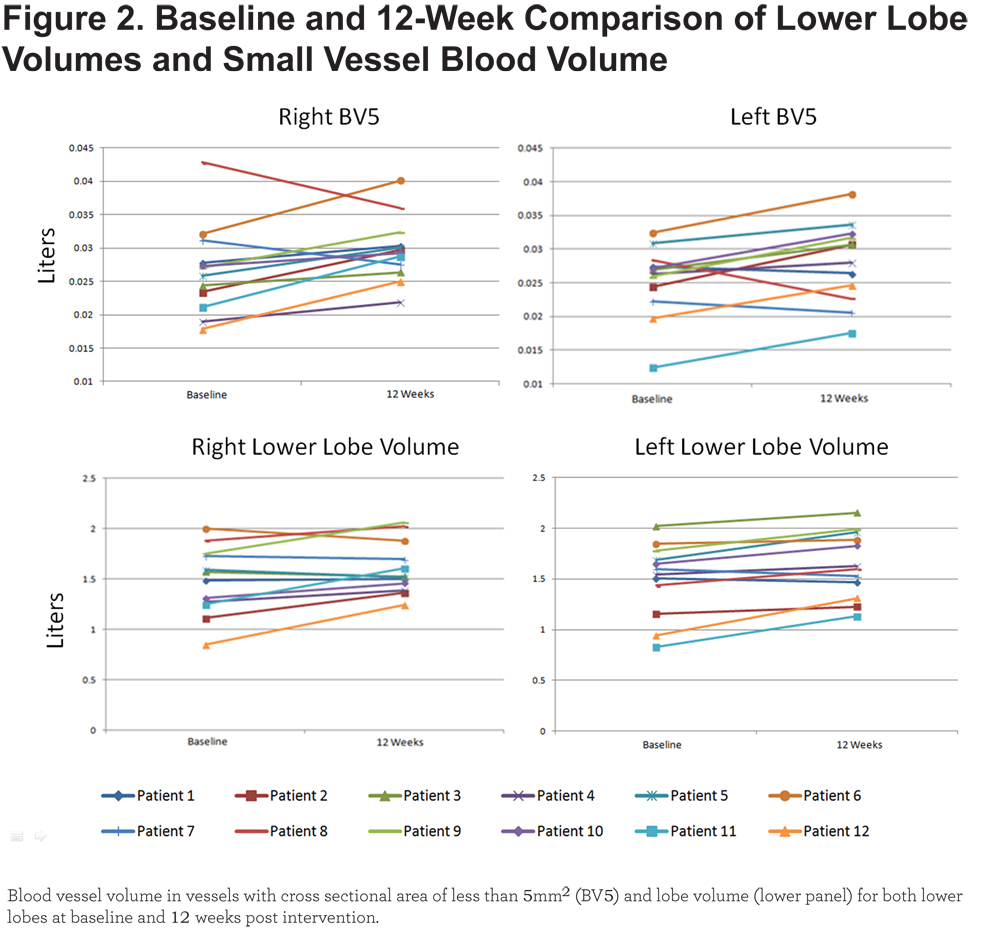
Relationships between changes in volume of different lung regions were examined. There was a statistically significant correlation between change in total lung volume and change in upper lobe (right and left combined) volume (R = 0.71; p = 0.01). There was, however, no correlation between change in lower lobe (right and left combined) volume and either change in upper lobe volume or change in total lung volume. In examining the relationships between changes in lobe volumes and CT-computed blood vessel volumes, a statistically significant correlation was found between the percent change in lower lobe volume and the percent change in small blood vessel volume in those lobes (R = 0.65; p = 0.02). There was no correlation between change in lower lobe volume and either large blood vessel volume (blood vessel cross sectional area >5mm2; BV>5) or TBV. There was no relationship between change in upper lobe volume and change in any lower lobe blood vessel volume. We compared the percentage change in BV5 to changes in FEV1 and no statistically significant correlation was found. However, the percentage change in BV5 when adjusted by the volume of the lobe (BV5/right lower lobe [RLL] volume) correlated with change in FEV1 (R = 0.69, p = 0.01).
Lastly, the RLL was examined separately (Figure 3) and arterial venous segmentation was performed in all baseline and follow-up scans. In this lobe, there were statistically significant changes in BV5 and TBV from baseline to 12 weeks post treatment (median 15.5%, p = 0.02 and 13.9%, p = 0.003, respectively). There was a trend toward increase in BV5 with increase in RLL volume post treatment (R = 0.49, p = 0.11). With AV segmentation, we found non-significant increases in BV5 in both the arterial and venous systems after treatment (median 12.8%, p = 0.2 and 6.3%, p = 0.27, respectively). Change in arterial and venous BV5 were directly related (R = 0.87) but neither changes in arterial nor venous BV5 had statistically significant correlations with RLL or right upper lobe (RUL) volume changes.
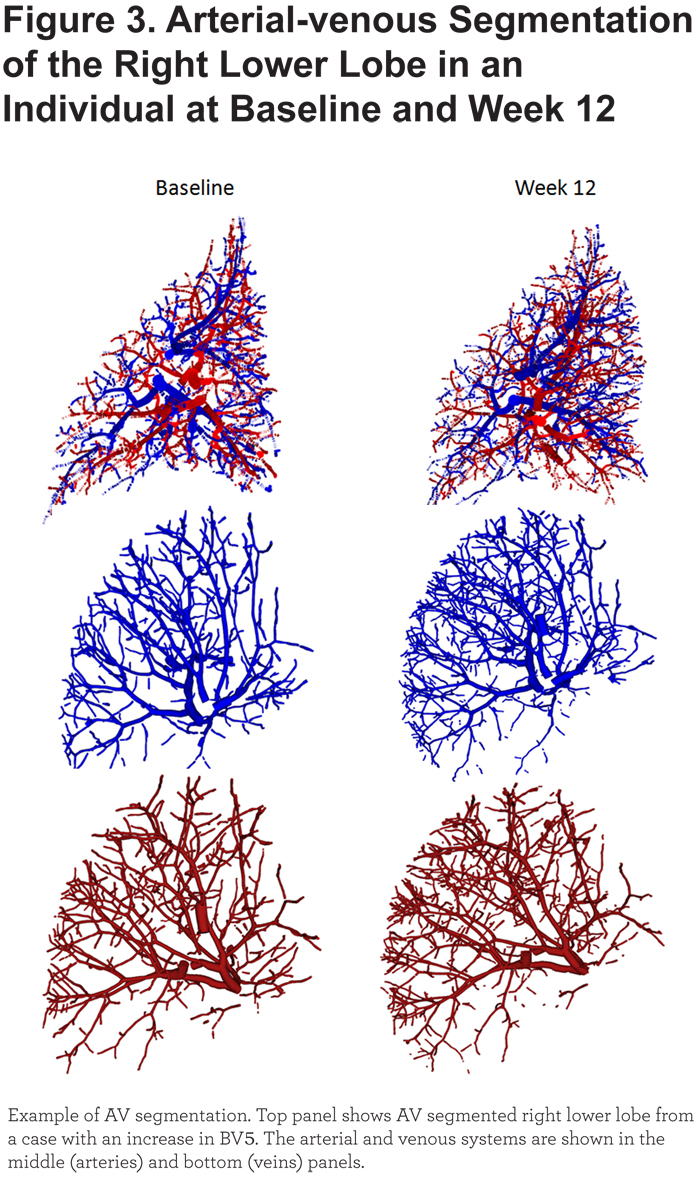
We further wanted to determine if change in venous BV5 was proportionate or in excess of the change in arterial BV5. The latter would suggest that vessels from the lower pressure intraparenchymal vessel system are more distensible. Since a correlation coefficient does not provide information on the slope of such a regression, we generated a scatter plot of change in venous BV5 versus change in arterial BV5 (Figure 4). A line of identity (slope of 1 with an intercept at the origin) would suggest that the change in arterial vessel volume was exactly proportional to the change in venous vessel volume. The slope of the line fit by least squares regression in these 12 individuals was 0.89.
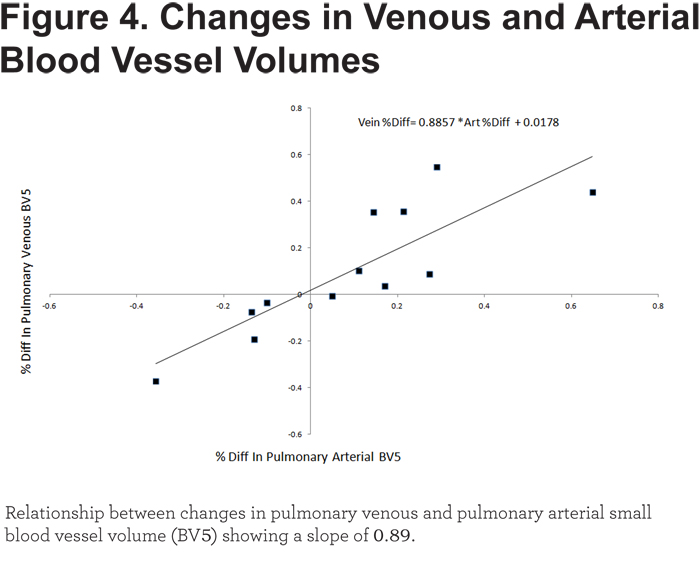
Discussion
Surgical and non-resectional lung volume reduction techniques have been demonstrated to improve lung and cardiac function. While the mechanism for the former has been investigated extensively, the basis for the latter is not as well defined but may be related to the mechanical interdependence of heart and lung. In our pilot study, we demonstrate that sealant-based endoscopic lung volume reduction had a measureable effect on the vasculature at sites distant from the treatment. Post procedural expansion of the lower lobes resulted in expansion of the blood vessel volume in those lobes. This effect appeared to be greatest in the distal vessels with a cross sectional area less than 5mm2 (i.e., BV5) and equally affected the arteries and veins.
Effective lung volume reduction procedures depend upon candidate sites for both treatment and preservation of lung remote to treatment sites. The physiologic response to such an intervention is predicated on the interdependence of the lobes; intervention at one site affects the pressure volume characteristics of the remaining tissue. While we found that reductions in upper lobe volume were predictive of changes in total lung volume, they were not related to changes in lower lobe volume, though this may be due to the small size of our cohort.
The blood vessel volume of the lower lobes increased in response to treatment. This change was most evident in the more distal vasculature (BV5) while there was little to no change in blood vessel volume of the larger more central vasculature. The measurements were automated and sources of measurement error are largely dependent on the quality of the CT scan including motion artifact and image acquisition modalities. We minimized these by excluding image pairs that did not match in baseline and follow up. The overall magnitude of the change was small in comparison of the total blood volume. However, the change in small vasculature visible by CT scan may indicate a larger change in the more distal microvasculature not visible by CT scan. Additionally, the change in perfusion which also must take into account flow, may be of a larger magnitude. Thus, we view this imaging-based biomarker as an indicator of the presence of increased blood volume but cannot estimate the magnitude of the impact on the microcirculation and overall perfusion without larger studies that measure these variables simultaneously.
A number of inter-related factors may contribute to increased small vessel density including changes in ventilation-perfusion matching and overall increase in perfusion,11,19 or recruitment of vessel due to increased lobe volume or decreased pressures with improved gas trapping. When examining the relationship of lobe and vessel volume we found that the change in lower lobe BV5 was related to the increase in volume of the lower lobes on follow-up CT scan; changes in the larger vessel volumes (BV>5 and TBV) were not. This preferential effect of treatment on distal blood vessel volume may be ascribed to small sample size or differential ability to measure changes in volumes in vessels of different size. Alternatively, these changes may reflect regional differences in the mural properties of the vasculature. The smaller intraparenchymal vessels may be more distensible than the central vessels that have larger CSAs. Thus, despite equal changes in transmural pressures post treatment, the smaller vessels may experience a greater proportional increase in diameter.
Because the pressures in the pulmonary venous system are very low, we hypothesized that venous vascular morphology might be more affected by decompression of the untreated lobe (i.e., veins may be more distensible). The slope of 0.89 in this regression model suggests that changes in venous and arterial BV5 are directly proportional. Small deviations from identity require a larger cohort to better define the relative effects of volume reduction on the arterial and venous circulatory systems.
Our study is limited by its retrospective nature (for example limiting the uniformity of imaging protocol) and small number of participants possessing the necessary imaging for intraparenchymal vascular reconstruction. We also do not have data pertaining to medical events (such as change in medications) that may have occurred between treatment and follow-up leaving the possibility that the observed change in vascular morphology was the result of other events and not treatment. Given our small sample size, such unrecorded events may introduce sufficient variability in our data to obscure potential trends of interest. Finally, the vascular reconstruction was limited by resolution of the CT scan which cannot objectively assess blood vessels with cross-sectional areas less than 1mm2. Based upon our initial findings that BV5 is more responsive to changes in volume than TBV, it is likely that examination of the change in distal or micro vascular blood vessel volume may be even more dramatic.
As mentioned, changes in small vessel volume in response to therapy may be an indicator of increased perfusion. The development of pulmonary hypertension in chronic obstructive pulmonary disease (COPD) involves long-term remodeling of the vasculature. Previous studies have shown the relationship with decreased vascular density and both the progression of emphysema15,18 and development of pulmonary hypertension.16 Thus, the change in small vascular volume in response to therapeutic interventions may be useful as a non-invasive imaging-based biomarker to assess the impact of interventions on the pulmonary vasculature which can be assessed relatively early in the course of therapy.
Conclusion
Endoscopic volume reduction using sealants leads to a decrease in the size of treated lobes and increases in both the size and blood vessel volume of untreated lower lobes. This increase in vascular volume correlated with change in volume of the untreated lobes and appears to be symmetric between arteries and veins, though the size of the cohort may limit detecting differences. In the future, larger, prospective studies with adequate imaging for vascular reconstruction along with concurrent pulmonary hemodynamic data would be necessary to confirm and extend the findings discussed here. The study of the morphology of the intraparenchymal pulmonary vasculature may serve as a method of assessing the effect of interventions on the pulmonary vascular system and help better define mechanisms and subphenotypes of pulmonary vascular disease in COPD.
Acknowledgements:
Approval for the procedure and data collection was granted by the internal review boards in Rabin and Soroka Medical Centers in Israel. This was a retrospective analysis of imaging and data collected during this study.
Declaration of Interest:
None of the authors have any conflicts of interest to declare regarding the contents of this article.